Innovative solder-free approach to diode bar assemblies
Packaging diode laser arrays. Why and how?
Edge-emitting semiconductor laser arrays (or laser bars) are surely the best-known and still the most widespread architecture of high power diode lasers (HPDLs). Under an electron-pumping scheme, these structures are nowadays capable of generating up to 500 W of CW optical power within an overall active material volume below 0.01 mm3. Although the electro-optical efficiency of such devices can easily surpass 50 % (especially for diode lasers based on GaAlAs and InGaAs), the portion of energy not converted into light is almost equally high. This translates into a huge amount of energy that must be dissipated from the laser device in the form of heat. Otherwise, the active medium would melt in a few microseconds. For this reason, heat dissipation is probably the major concern in HPDL mounting technology. The heat generated is firstly transmitted by conduction to the surrounding substrate volume, which is normally between 1 and 4 mm3. However, the heat rate is too high for such a small volume, and further dissipating steps are mandatory in order to spread the heat through a larger volume of material before it can be finally removed by the environment (typically forced convection towards air or water). Moreover, this needs to be a fast enough process to avoid excessive temperature rise at the active medium. Copper is the natural choice for such application, since it allows excellent electron conductivity, and shows the second largest thermal conductivity among all metals (k~385 W·m-1·K-1). It is slightly surpassed by silver (k ~ 405 W·m-1·K-1), but silver is not an option for obvious commercial reasons. From the beginning of semiconductor laser technology, copper has been used as the preferred sub-mount material, but then the next technical challenge appeared: how to establish a proper interface between the laser bar and the copper heat sink? Soldering was the first answer, and it is still the most adopted technology. But soldering brings along several inherent problems…
The interface material chosen for soldering purposes must have a melting temperature well below the melting points of both the laser bar and the heat sink. Besides, it must be high enough to guarantee thermal and mechanical stability over the operation temperature of the laser diode (typically between 15 ºC and 80 ºC). Indium was the first material employed with this purpose (Tmelt ~ 157 ºC). However, the soldering process implies to create a solid joint between the laser bar and the copper heat sink around the indium melting temperature, and since the three material involved show different coefficients of thermal expansion (CTE), the device will always present a residual stress when it cools down to room temperature.
Smile in laser diode arrays. A serious problem
One of the most important implications of this is the appearance of the smile phenomena, which means that bar suffers a curvature, causing the emitters within the array not lasing perfectly parallel to the horizontal axis. Instead, a separation in height ranging from 2 μm to 5 μm (typically) appears from the bottom to the top emitter (see Figure 1). This distance is usually the best measure to define smile magnitude. The smile in laser bars is critical whenever external resonator configurations are required or simply if maximum brightness is demanded from the fast axis.
Figure 1.
Theoretical emission intensity pattern after fast axis collimation and
slow axis imaging of a 19-emitter laser bar. Top image corresponds to a
smile-free laser bar. The two bottom images correspond to two different
kind of smile effect.
External resonator configurations
To
build an external resonator using a laser bar is an appealing strategy
used mainly to increase power brightness or spectral brightness of the
laser bar itself. High spatial brightness (W·cm-2·sr) is
pursued in high power diode lasers when industrial applications like
metal cutting, drilling or soldering come into play. Besides, high
spectral brightness (W· cm-2·sr·nm-1) and low wavelength thermal shift (nm·K-1)
are typically related to solid state laser pumping applications. In the
case of power brightness, the external feedback is created with a
reflective diffraction Bragg grating (see Figure 2).
The result of such approach is the effective spatial superimposition of
all the laser beams outcoming from the laser bar, as if all the
intensity was coming from a single emitter within the laser bar. As a
consequence, the spatial brightness is increased by an order of
magnitude. However, this can be achieved at the expense of enlarging the
emission bandwidth (lower spectral brightness) and admitting a certain
percentage of power and optical losses (ranging from overall 20 % to
40 % reduction in power w.r.t. free beam operation). Inherent (and
unavoidable) optical losses are attributed to the efficiency of the
diffraction grating and the transmission of the lenses. But most
importantly, optical losses are also affected by how the laser beam hits
back the emitter after part of its intensity is bounced back at the
outcoulping mirror (feedback emission from the external resonator). The
larger the smile effect, the higher the losses (see Figure 3 and Figure 4).
Figure 2. Basic
scheme illustrating the spectral beam combining principle. Spatially
separated emitters emitting at slightly different wavelengths impinge
the diffraction grating at different incident angles. However, the
diffracted angle is common to all of them (different colours are used
here just to illustrate the difference in wavelength but they are not
representative of the wavelength itself).
Figure 3. Most
of the emitters in a laser bar with smile are partially or totally out
of the plane defined by the fast axis and the optical axis of the
system. This results in a partial lack of optical feedback in an
external resonator configuration (most of the emitted and feedback beams
are partly or totally non-coincident).
Figure 4.
The emitters of a laser bar with no smile are contained within the plane
defined by the fast axis and the optical axis of the system. As a
consequence, the emitted laser beams and its partially-reflected
counterpart (feedback) are spatially coincident in an external resonator
configuration.
Figure 4. The emitters of a laser bar with no smile are contained within the plane defined by the fast axis and the optical axis of the system. As a consequence, the emitted laser beams and its partially-reflected counterpart (feedback) are spatially coincident in an external resonator configuration.
In the case of spectral brightness
improvement, usually volume Bragg gratings (VBGs) are placed in front of
a fast axis collimated laser bar. Again, an external feedback is
required by the Bragg grating, which now acts narrowing and “locking”
the emission wavelength of the whole laser bar. The effect is a
remarkable reduction in the wavelength shift w.r.t. temperature, from
0.3 nm·K-1 to less than 0.08 nm·K-1, in a similar
way as it happens with distributed feedback lasers (either applied on
the diode structure itself or by an optical fibre coupled to it). The
question here is that having a laser diode array, the absence of smile
is crucial in order to have uniform feedback on each emitter, especially
if we consider that the optical elements are common to all the emitters
(see Figure 4).
Fast axis brightness
Laser emission in the fast axis is inherently almost diffraction-limited (M2~1).
This represents an outstanding advantage in many applications where
maximum brightness is demanded along a line-shaped laser spot. Such is
the case of offset laser printing (computer-to-print machines).
Nevertheless, the presence of smile effect in a laser bar can
reduce overall fast axis brightness easily between 50 % and 80 %. The
main reason is that the apparent height of a laser source in the fast
axis gets increased proportionally to the smile. From a
practical perspective, the consequences of placing a fast axis
collimator (FAC) lens in front of the laser bar is either obtaining a
higher residual divergence or a larger focused spot (see Figure 6).
Figure 5. Near-field representation of a 10-emitter laser bar with no smile (top) and with 3 μm
smile effect (bottom). The apparent size (represented by the dashed
frames below) is enlarged in the fast axis due to the smile.
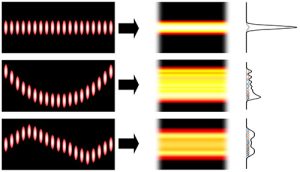
Figure 6.
Different smile patterns (left) yield different fast axis intensity
profiles under fast axis collimation (middle). The far field intensity
profile along the fast axis is the result of superimposing as many
line-shaped spots as emitters within the laser bar (right).
Smile suppression. What are the alternatives?
Despite the challenge, the HPDL industry found ways to overcome smile
effect. The most extended solution is called “hard-soldering”. This
consists of using a AuSn alloy as the interface material, and CuW as the
heat sink.
This way, the CTE of the semiconductor, heat sink metal and soldering
interface are much closer, the joint is even more reliable than in the
case of indium, and the smile effect is minimized. However,
nothing here is for free. CuW shows a remarkable reduction of thermal
conductivity compared to copper (around 50 % lower), a far lower degree
of machinability and far higher cost. As a consequence, usually CuW is
used just as an intermediate volume between the laser bar and the actual
heat sink, which is made of copper indeed. This adds further thermal
resistance jumps to the laser diode package. In the end, the advantages
of such an approach are restricted to a few set of applications.
So,
what can be done apart from indium and hard-soldering? The ultimate
alternative has been shown and demonstrated by Monocrom for the last 20
years, and it relies on an extremely simple concept: mechanical pressure
(ClampingTM). However, as the reader might guess, conceptual
simplicity does not necessarily imply easy engineering, and for this
reason Monocrom remains as the only company able to bring such a
revolutionary approach to laser diode packaging. ClampingTM
technology relies (mainly) on a superior surface finish of the copper
heat sink, and the establishment of direct thermal and electrical
contact with the laser bar, enhanced by the application of mechanical
force. While soldered bars are contacted to the p-side by the heat sink
(anode) and wire-bonding to the n-side (cathode), clamped bars are
“sandwiched” from both sides by bulky heat sinks acting as the anode and
cathode.
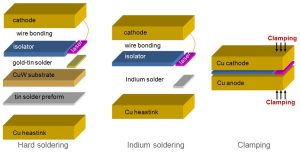
Figure 7. Comparison between the most common soldering approaches for laser bar packaging and ClampingTM technology.
The advantages of ClampingTM are numerous:
– Cold process. No residual stress is caused by dissimilar CTE, so the smile is limited to the surface flatness achieved in the heat sink (typically μm over 1 cm2).
–
Minimum thermal resistance jumps. Heat is directly evacuated from the
laser bar to the heat sink. Only the contact resistance between copper
and the semiconductor material must be accounted from a technical point
of view.
–
Heat is dissipated from both p and n side, adding an extra path for
heat dissipation. Additionally, this allows the use of water/glycol
cooling channels machined on both electrodes in the case of CW and high
duty QCW operation. Likewise, this fact relaxes the requirement for
micro-channel cooling. Millimetre-sized channels can be used instead,
which are less corrosion sensitive and consequently allows to minimise
maintenance.
–
Simplicity and cost reduction. There is no interface material involved
and no soldering equipment. Mechanical force is applied by the operator
by means of a stainless steel screw. The key is on the perfect surface
finish of the copper heat sink (but of course this is not the only one).
–
Superior performance in pulsed mode. The lack of horizontal residual
stress eliminates the fatigue suffered by the joint and the laser bar
during successive on/off cycles, enhancing the service life of the
device.
Figure 8.
Typical intensity profile of the individual emitters (fast axis
collimation plus slow axis imaging) within a soldered laser bar (left)
and a clamped laser bar (right).
In conclusion, ClampingTM
can be seen as a smart solution to a challenging packaging problem,
which has been successfully implemented at Monocrom as part of the
standard manufacturing process.
_________________
[1] CTEIn = 33 μm·m-1·K-1; CTECu = 17 μm·m-1·K-1; CTEGaAs = 5 μm·m-1·K-1
[2] CTEAu(80)Sn(20) = 16 μm·m-1·K-1; CTECuW = 5-9 μm·m-1·K-1
[/vc_column_text][/vc_column][/vc_row]